When light moves from one material into another, it bends — like how a partially submerged object appears distorted under water when viewed from above. What if, instead of bending, a material could change the light so much that the material was no longer visible at all?
In a study published in Physical Review A, University of Wisconsin–Madison researchers have shown for the first time that a similar response can be obtained and manipulated in naturally-occurring materials. The findings have implications from the development of “perfect” lenses for improved microscopy to Harry Potter-esque invisibility cloaks.
Visible light is made of both magnetic and electric fields, and the refractive index of a material — how much it bends the light — is determined by how the material interacts with those two fields. Nearly all materials we encounter in everyday life, though, interact entirely with light’s electric field.
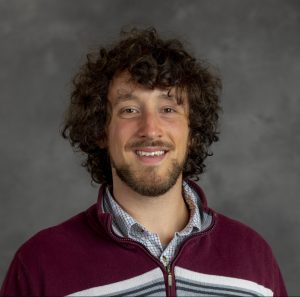
Researchers have spent the past two decades developing artificial materials that more strongly interact with light’s magnetic field by manipulating the refractive index. With a strong enough response, the material could eventually have a negative refractive index, leading to unique optical properties. However, the response in synthetic materials is limited by the size of their repeating units. A naturally-occurring crystal that has much smaller unit cells is likely a better choice.
“Part of producing a negative refractive index is that the material needs to have a strong response to both electric and magnetic fields, so the big challenge is getting that magnetic response in natural materials,” explains Zach Buckholtz, a graduate student in UW–Madison physics professor Deniz Yavuz’s group and lead author of the study. “A few years ago, we published a paper showing that the crystal we’re working with has a magnetic response, and in this study, we were able to manipulate the response.”
The natural material Buckholtz is working with is a silicon-based crystal, which in general is optically ordinary, except that it has been “doped” with the rare earth metal Europium. Rare earth metals are unique in that they contain an abundance of electrons in the atoms’ outer energy shells. Those electrons can then work together to create a bigger magnetic response, but only if they are all in tune with each other.
“If you have some magnetic response and a much larger electric response to light, you can connect those two responses,” Buckholtz says. “To get to a negative refractive index from there, you have to set up coherences between the energy levels, meaning you have to make sure all those energy levels are oscillating together.”
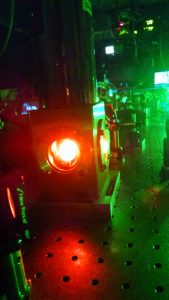
To show they can manipulate the magnetic response, Buckholtz and Yavuz did two things. First, because the crystal is a mix of ions with slightly different electron responses, they needed to set up their experimental system to select for one class of ion. This uniformity allows for a cleaner interpretation of the results.
“We send a laser into the crystal, and then measure how much of the light is transmitted. But because the crystal isn’t perfect, instead of seeing a narrow peak for the transitions, you’ll see a really broad transition,” Buckholtz explains. “So, we do this procedure known as spectral hole burning to clear out the ions we don’t want and then we’ll be left with just one transition, which is necessary to move on to experiments that involve coherence.”
Next, they wanted to show if they could increase the magnetic response. To do so, they needed to take those selected ions, put them in coherence, and then measure the response compared to ions not in coherence. In these experiments, they shined one (a probe beam) or two (probe and coupling beam) wavelengths of laser at the ions. Both lasers excite electrons in the ions to a higher-energy state, and the scientists can again measure how might light is transmitted through the crystal as a readout of the electron transitions.
“With just the probe beam, we see just the normal transition, and that’s what we did in our previous study. But with the coupling beam added in, it connects and adds another transition state in there,” Buckholtz says. “If those states are in coherence, they cancel each other out, and we see that effect as a peak in transmitted light, which means the index of refraction is going toward zero.”
Buckholtz notes that the magnetic response they see is not yet large enough to produce the materials with interesting new optical properties they are hoping for. Still, he says, this work provides a path forward to continue manipulations to improve the response, such as investigating different rare earth metals.
“We have a magnetic response, we can set up coherence, and we can manipulate the response,” Buckholtz says. “Now, we want to increase the scale of the response to with a goal of eventually making the refractive index below zero.”