Qubits are notoriously more prone to error than their classical counterparts. While superconducting quantum computers currently use on the order of 100 to 1000 qubits, an estimated one million qubits will be needed to track and correct errors in a quantum computer designed for real-world applications. At present, it is not known how to scale superconducting qubit circuits to this size.
In a new study published in PRX Quantum, UW–Madison physicists from Robert McDermott’s group developed and tested a new superconducting qubit architecture that is potentially more scalable than the current state of the art. Control of the qubits is achieved via “Single Flux Quantum” (SFQ) pulses that can be generated close to the qubit chip. They found that SFQ-based control fidelity improved ten-fold over their previous versions, providing a promising platform for scaling up the number of qubits in a quantum array.
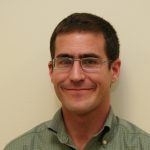
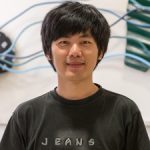
The architecture involves a sandwich of two chips: one chip houses the qubits, while the other contains the SFQ control unit. The new approach suppresses the generation of quasiparticles, which are disruptions in the superconducting ground state that degrade qubit performance.
“This structure physically separates the two units, and quasiparticles on the SFQ chip cannot diffuse to the quantum chip and generate errors,” explains Chuan-Hong Liu, PhD ’23, a former UW–Madison physics graduate student and lead author of the study. “This design is totally new, and it greatly improves our gate fidelities.”
Liu and his colleagues assessed the fidelity of SFQ-based gates through randomized benchmarking. In this approach, the team established operating parameters to maximize the overall fidelity of complex control sequences. For instance, for a qubit that begins in the ground state, they performed long sequences incorporating many gates that should be equivalent to an identity operation; in the end, they measured the fraction of the population remaining in the ground state. A higher measured ground state population indicated higher gate fidelity.
Inevitably, there are residual errors, but the reduced quasiparticle poisoning was expected to lower the error rate and improve gate fidelities — and it did.
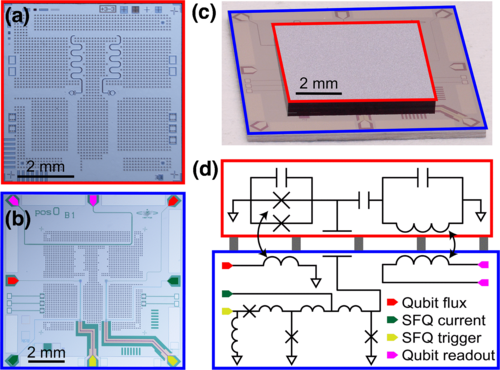
“Most of the gates had 99% fidelity,” Liu says. “That’s a one order of magnitude reduction in infidelity compared to the last generation.”
Importantly, they showed the stability of the SFQ-based gates over the course of a six-hour experimental run.
Later in the study, the researchers investigated the source of the remaining errors. They found that the SFQ unit was emitting photons with sufficient energy to create quasiparticles on the qubit chip. With the unique source of the error identified, Liu and his colleagues can develop ways to improve the design.
“We realized this quasiparticle generation is due to spurious antenna coupling between the SFQ units and the qubit units,” Liu says. “This is really interesting because we usually talk about qubits in the range of one to ten gigahertz, but this error is in the 100 to 1000 gigahertz range. This is an area people have never explored, and we provide a straightforward way to make improvements.”
This study is a collaboration between the National Institute of Standards and Technology, Syracuse University, Lawrence Livermore National Laboratory, and UW–Madison.
This work was funded in part by the National Science Foundation (DMR-1747426); the Wisconsin Alumni Research Foundation (WARF) Accelerator; Office of the Director of National Intelligence, Intelligence Advanced Research Projects Activity (IARPA-20001-D2022-2203120004); and the NIST Program on Scalable Superconducting Computing and the National Nuclear Security Administration Advanced Simulation and Computing Beyond Moore’s Law program (LLNL-ABS-795437).