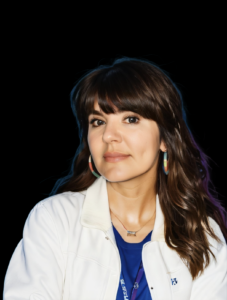
Melinda Soares-Furtado, an observational astronomer, joined the UW–Madison faculty this fall on a joint appointment in the astronomy and physics departments. She earned her undergrad degree at UC-Santa Cruz, then her doctorate in astrophysical sciences at Princeton. In 2020, she began a postdoc apppointment in UW–Madison astronomy, where she subsequently was awarded a NASA Hubble Postdoctoral Fellowship.
Please give an overview of your research.
I’m interested in stars and the planets that orbit them. So far, here at Madison, my team has detected and characterized two young, nearby planets. I want to know as much as possible about the worlds we discover, and part of that investigation includes knowing as much as possible about the stars the planet orbits. We have lines of research focused on the stellar age, its local environment (is it isolated or moving with a large collection of stars?), and its activity. Is the planet orbiting the star in a docile, stable environment or one that makes it more challenging to retain an atmosphere? How can we use follow-up observations both on the ground and with space-based facilities to get new insights into these worlds? Can instruments like HST and JWST offer a glimpse of the planet’s atmospheric evolution? Given the ever-expanding number of worlds we have discovered over the past three decades, how unusual is our own Solar System?
What are one or two main projects your group will work on first?
I have a broad range of research interests, so one or two main projects is sort of hard to narrow down. Right now, I’m most excited about the young worlds we have found in the Solar Neighborhood and the added context we can get with additional observations. I’d like to know the mass of the mini-Neptune-sized planet we recent found and here at UW-Madison, we have access to the institutional resources that will allow us to make this measurement! This planet is compelling, because it is found at the upper edge of a distribution known as the “radius valley”. The mass can help us understand the eventual fate of this young planet orbiting an active M dwarf star. I’m also interested to see what we learn with JWST and HST about an Earth-sized planet we found orbiting a Sun-like star. Will we see signs of atmospheric outgassing?
Putting on my stellar astronomer hat, I’m also really intrigued by what more we can learn about stars and ways in which we can better estimate their ages and evolutionary histories. Again, here at UW-Madison, our institutional access makes it possible to probe some of these mysteries in impactful ways — largely due to our access to WIYN/NEID, which offers high-precision measurements of a star’s shifting spectral lines.
What attracted you to Madison and the University?
I was drawn to the University of Wisconsin–Madison for its exceptional research environment and the wealth of opportunities available for collaboration. The Department of Astronomy is not only broad in its research pursuits — it is also notably collegiate, fostering a collaborative and supportive atmosphere among faculty, students, and staff. Access to cutting-edge facilities such as WIYN/NEID, SALT, and NOEMA was a strong attraction, as these instruments enable a range of high-impact research opportunities, from precise stellar characterization to molecular gas studies.
I was also excited to join the Wisconsin Center for Origins Research (WiCOR) collaboration. The Wisconsin Center for Origins Research (WiCOR) is a multidisciplinary center at UW-Madison designed to unite researchers from diverse scientific departments, including astronomy, chemistry, geoscience, and biology, to study the origins of life in the universe. Recently established with a dedicated research space, WiCOR not only focuses on cutting-edge projects — such as investigating potentially habitable exoplanets with the James Webb Space Telescope—but also emphasizes public outreach and educational initiatives, making it a leader in origins research and science communication.
What is your favorite element and/or elementary particle?
I think everyone in the department knows I have a fondness for lithium! As a PhD student, I worked on the signatures of stars that ingest their planetary companions, finding that lithium excess can sometimes be observed. I predicted which stars which show such an enhancement, and this was verified in large abundance surveys a year later. Lithium is a useful flag for engulfment, because it is readily destroyed in the interiors of forming stars, but never reaches temperatures required for destruction in planets. It therefore can be one to two orders of magnitude higher in these less massive bodies. If a star ingests a planet later in its evolution, that signature is sometimes observable! I like to hunt for such lithium-rich stars and then explore other aspects of their chemistry to better understand the cause of their enrichment.
What hobbies and interests do you have?
I like to garden, read, and spend time with my family. I often hike the Grady Tract Loop not far from my home. I have an app I use to identify plants, fungi, and wildlife. My daughter and I like to use it when we go on walks together. I have a fondness for photography. During my undergraduate years, I worked with my sister as a family photographer in California. These days I mostly photograph plants and landscapes. I also love to dance cumbia, salsa, and bachata. I danced often when I was growing up and even spent some time on a salsa choreography team in San Jose, California. I also collect and read vintage textbooks — most of my favorites are from the 1930s.