Neutrinos are weakly interacting particles that are able to travel undeflected through the cosmos. The IceCube Neutrino Observatory and the KM3NeT Astroparticle Research with Cosmics in the Abyss (ARCA) telescope (still under construction) are cubic-kilometer-scale neutrino telescopes that search for the sources of these astrophysical neutrinos in hopes of uncovering the origin of ultra-high-energy cosmic [...]
Read the full article at: https://wipac.wisc.edu/probing-the-connection-between-the-highest-energy-astrophysical-neutrinos-and-ultra-high-energy-cosmic-rays/Astrophysics
Baha Balantekin honored at neutrino astrophysics workshop
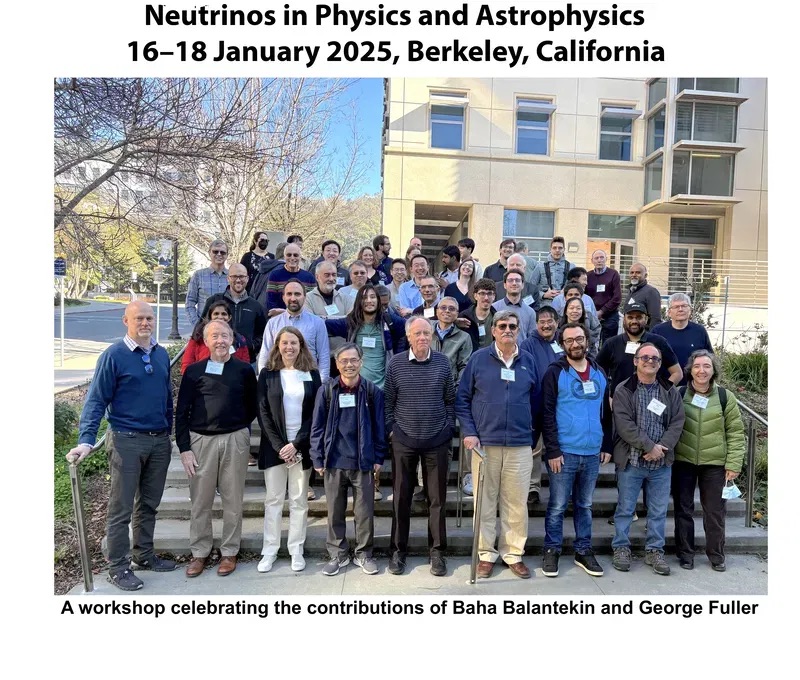
The illustrious career of Baha Balantekin, the Eugene P. Wigner professor of physics at UW–Madison, was celebrated recently at the Neutrinos in Physics and Astrophysics Workshop through the Network for Neutrinos, Nuclear Astrophysics, and Symmetries (N3AS) Physics Frontier Center.
Balantekin works at the intersection of nuclear physics, particle physics, and astrophysics. For much of his career, he has studied theoretical aspects of neutrinos originating in the Sun, supernovae, or neutron star mergers. He has helped pioneer the field known as neutrino astronomy.
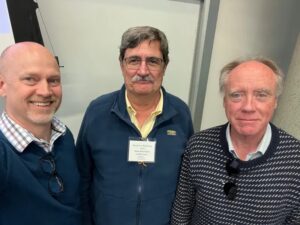
“Even just a few decades ago, if you said ‘neutrino astronomy,’ most physicists would have snickered. That’s because astronomy is about observations and neutrinos are almost impossible to detect,” says John Beacom, PhD ’97, distinguished professor of physics and astronomy at the Ohio State University. “But, over time, physicists have helped to make this seemingly impossible field into something real and vibrant. The observations of astrophysical neutrinos that have been made have been essential to understanding our Sun, supernovae, and distant galaxies.”
Balantekin and George Fuller, a distinguished professor of physics at the University of California, San Diego, have helped lead the field of neutrino astrophysics through both their scientific work and their mentoring of junior scientists. To honor both scientists’ significant and ongoing contributions to the field, three of their former students organized the workshop: Beacom, a former student of Balantekin’s, and Fuller’s former students Gail McLaughlin, distinguished university professor of physics at North Carolina State University and Yong Zhong Qian, professor of physics and astronomy at the University of Minnesota. The event was held Jan 16-18 at the University of California, Berkeley.
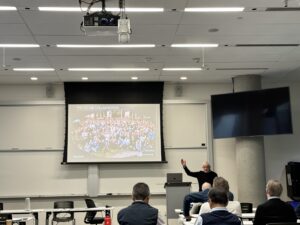
Francis Halzen, a current colleague of Balantekin’s at UW–Madison, was one of the speakers. Other attendees included UW–Madison physics professor Pupa Gilbert and professor emerit Sue Coppersmith.
John Beacom and Pupa Gilbert contributed significantly to this story
Dan McCammon awarded Distinguished Career Prize
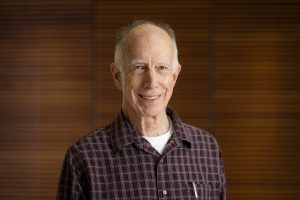
Congrats to Prof. Dan McCammon for earning the Distinguished Career Award from The American Astronomical Society’s (AAS) High Energy Astrophysics Division (HEAD) for his pioneering work on the development of microcalorimeters that has led to breakthroughs in X-ray astronomy and on soft diffuse X-ray background.
The HEAD Distinguished Career Prize is awarded at the time of the Division Meeting to recognize an individual high-energy astrophysicist who has made outstanding contributions to the field of high energy astrophysics throughout their career. Outstanding contributions include a body of important research results (observational, theoretical or experimental) which have led to ground-breaking results in high-energy astrophysics, and/or a career of mentorship to a new generation of high-energy astrophysicists, especially if this mentorship helped to support under-represented or under-resourced scientists and increased the diversity of the HEA community. The winner gives an invited talk at the Divisional Meeting in the award year. The prize carries a cash award of $1500.
AAS announced many 2025 prizes today; the full list can be found at their website.
This post is adapted from the AAS news release and website linked within the text.
Welcome, Prof. Melinda Soares-Furtado!
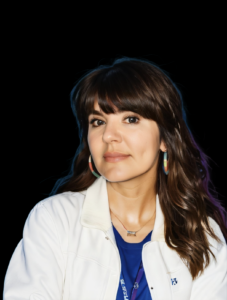
Melinda Soares-Furtado, an observational astronomer, joined the UW–Madison faculty this fall on a joint appointment in the astronomy and physics departments. She earned her undergrad degree at UC-Santa Cruz, then her doctorate in astrophysical sciences at Princeton. In 2020, she began a postdoc apppointment in UW–Madison astronomy, where she subsequently was awarded a NASA Hubble Postdoctoral Fellowship.
Please give an overview of your research.
I’m interested in stars and the planets that orbit them. So far, here at Madison, my team has detected and characterized two young, nearby planets. I want to know as much as possible about the worlds we discover, and part of that investigation includes knowing as much as possible about the stars the planet orbits. We have lines of research focused on the stellar age, its local environment (is it isolated or moving with a large collection of stars?), and its activity. Is the planet orbiting the star in a docile, stable environment or one that makes it more challenging to retain an atmosphere? How can we use follow-up observations both on the ground and with space-based facilities to get new insights into these worlds? Can instruments like HST and JWST offer a glimpse of the planet’s atmospheric evolution? Given the ever-expanding number of worlds we have discovered over the past three decades, how unusual is our own Solar System?
What are one or two main projects your group will work on first?
I have a broad range of research interests, so one or two main projects is sort of hard to narrow down. Right now, I’m most excited about the young worlds we have found in the Solar Neighborhood and the added context we can get with additional observations. I’d like to know the mass of the mini-Neptune-sized planet we recent found and here at UW-Madison, we have access to the institutional resources that will allow us to make this measurement! This planet is compelling, because it is found at the upper edge of a distribution known as the “radius valley”. The mass can help us understand the eventual fate of this young planet orbiting an active M dwarf star. I’m also interested to see what we learn with JWST and HST about an Earth-sized planet we found orbiting a Sun-like star. Will we see signs of atmospheric outgassing?
Putting on my stellar astronomer hat, I’m also really intrigued by what more we can learn about stars and ways in which we can better estimate their ages and evolutionary histories. Again, here at UW-Madison, our institutional access makes it possible to probe some of these mysteries in impactful ways — largely due to our access to WIYN/NEID, which offers high-precision measurements of a star’s shifting spectral lines.
What attracted you to Madison and the University?
I was drawn to the University of Wisconsin–Madison for its exceptional research environment and the wealth of opportunities available for collaboration. The Department of Astronomy is not only broad in its research pursuits — it is also notably collegiate, fostering a collaborative and supportive atmosphere among faculty, students, and staff. Access to cutting-edge facilities such as WIYN/NEID, SALT, and NOEMA was a strong attraction, as these instruments enable a range of high-impact research opportunities, from precise stellar characterization to molecular gas studies.
I was also excited to join the Wisconsin Center for Origins Research (WiCOR) collaboration. The Wisconsin Center for Origins Research (WiCOR) is a multidisciplinary center at UW-Madison designed to unite researchers from diverse scientific departments, including astronomy, chemistry, geoscience, and biology, to study the origins of life in the universe. Recently established with a dedicated research space, WiCOR not only focuses on cutting-edge projects — such as investigating potentially habitable exoplanets with the James Webb Space Telescope—but also emphasizes public outreach and educational initiatives, making it a leader in origins research and science communication.
What is your favorite element and/or elementary particle?
I think everyone in the department knows I have a fondness for lithium! As a PhD student, I worked on the signatures of stars that ingest their planetary companions, finding that lithium excess can sometimes be observed. I predicted which stars which show such an enhancement, and this was verified in large abundance surveys a year later. Lithium is a useful flag for engulfment, because it is readily destroyed in the interiors of forming stars, but never reaches temperatures required for destruction in planets. It therefore can be one to two orders of magnitude higher in these less massive bodies. If a star ingests a planet later in its evolution, that signature is sometimes observable! I like to hunt for such lithium-rich stars and then explore other aspects of their chemistry to better understand the cause of their enrichment.
What hobbies and interests do you have?
I like to garden, read, and spend time with my family. I often hike the Grady Tract Loop not far from my home. I have an app I use to identify plants, fungi, and wildlife. My daughter and I like to use it when we go on walks together. I have a fondness for photography. During my undergraduate years, I worked with my sister as a family photographer in California. These days I mostly photograph plants and landscapes. I also love to dance cumbia, salsa, and bachata. I danced often when I was growing up and even spent some time on a salsa choreography team in San Jose, California. I also collect and read vintage textbooks — most of my favorites are from the 1930s.
UW–Madison joins new NSF-Simons AI Institute for the Sky
This post is modified from the original news story from Northwestern University
A large multi-institutional collaboration— led by Northwestern University and including UW–Madison physics professors Keith Bechtol, Kyle Cranmer, and Moritz Münchmeyer — has received a $20 million grant to develop and apply new artificial intelligence (AI) tools to astrophysics research and deep space exploration.
Jointly funded by the National Science Foundation (NSF) and the Simons Foundation, the highly competitive grant will establish the NSF-Simons AI Institute for the Sky (SkAI, pronounced “sky”). SkAI is one of two National AI Research Institutes in Astronomy announced today. Northwestern astrophysicist Vicky Kalogera is principal investigator of the grant and will serve as the director of SkAI. Northwestern AI expert Aggelos Katsaggelos is a co-principal investigator of the grant.
The new institute will unite multidisciplinary researchers to develop innovative, trustworthy AI tools for astronomy, which will be used to pursue breakthrough discoveries by analyzing large astronomy datasets, transform physics-based simulations and more. With unprecedentedly large sky surveys poised to launch, including from the Vera C. Rubin Observatory in Chile, astronomers will require smarter, more efficient tools to accelerate the mining and interpretation of increasingly large datasets. SkAI will fulfill a crucial role in developing and refining these tools.
HAWC detection of an ultra-high-energy gamma-ray bubble around a microquasar
This story is adapted from the HAWC Collaboration press release. Microquasars—compact regions surrounding a black hole with a mass several times that of its companion star—have long been recognized as powerful particle accelerators within our galaxy. The enormous jets spewing out of microquasars are thought to play an important role in the production of galactic cosmic rays, although [...]
Read the full article at: https://wipac.wisc.edu/hawc-detection-of-an-ultra-high-energy-gamma-ray-bubble-around-a-microquasar/Baha Balantekin wins 2025 APS Bethe Prize
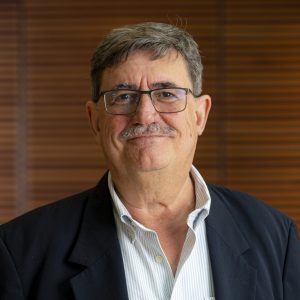
Congrats to Prof. Baha Balantekin on winning the American Physical Society’s 2025 Hans A. Bethe prize!
The Bethe prize is awarded to recognize outstanding work in theory, experiment or observation in the areas of astrophysics, nuclear physics, nuclear astrophysics, or closely related fields. Balantekin won “for seminal contributions to neutrino physics and astrophysics — especially the neutrino flavor transformation problem — both for solar neutrinos and the nonlinear supernova environment.”
Balantekin works at the intersection of particle physics, nuclear physics, and astrophysics. For much of his career, he has studied theoretical aspects of neutrino transport originating in the Sun, supernovae, or neutron star mergers.
“The concepts (I brought to the field) were marrying neutrino physics with many-body physics,” Balantekin says. “Of course, incorporating many-body aspects is common in condensed matter and nuclear physics, but it’s not as common in environments studied in astrophysics.”
Several fundamental astrophysical processes produce neutrinos as byproducts, and scientists have been studying neutrino origins and patterns for decades. Detecting the Sun’s neutrinos can reveal insights into its nuclear reactions, for example, and detecting neutrinos from core collapse supernovae can reveal insights into the early universe.
Balantekin’s early research was on the theory of neutrino transport from the Sun. He has also been studying core collapse supernovae, the result of a star running out of nuclear fuel. During collapse, a very hot star cools very quickly, emitting neutrinos on the order of 10^58.
“A number of that magnitude means you can no longer ignore the neutrino-neutrino interactions,” Balantekin says. “And then it becomes a very interesting many-body problem, where you have two-body interactions between neutrinos, and the propagation, and then it becomes a very complex problem.”
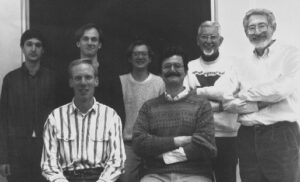
To describe this problem, has more recently begun using techniques from quantum information science to study entanglement of neutrinos with each other and to look at the signatures of such interactions and how they might contribute to heavy element formation.
The Bethe Prize was awarded solely to Balantekin, but he says he would not have won it without his collaborators over the years.
“You don’t do work in a vacuum,” Balantekin says. “I’ve worked with a lot of very talented young people. I would like to acknowledge first not only my graduate students at Wisconsin, but also the Fellows who came from the N3AS Physics Frontier Center we have. And the people I collaborate with around the world. We also have colleagues here in the department like Sue Coppersmith and Mark Saffman who contributed many ideas.”
The Bethe prize consists of $10,000 and a certificate citing the contributions made by the recipient. It is presented annually.
Entangled neutrinos may lead to heavier element formation
Elements are the building blocks of every chemical in the universe, but how and where the different elements formed is not entirely understood. A new paper in The Astrophysical Journal by University of Wisconsin–Madison physics professor Baha Balantekin and colleagues with the Network for Neutrinos, Nuclear Astrophysics, and Symmetries (N3AS) Physics Frontier Center, shows how entangled neutrinos could be required for the formation of elements above approximately atomic number 140 via neutron capture in an intermediate-rate process, or i-process.
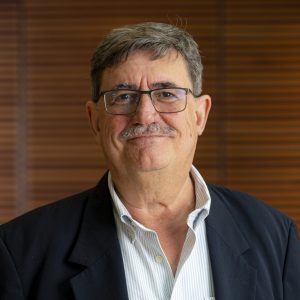
Why it’s important
“Where the chemical elements are made is not clear, and we do not know all the possible ways they can be made,” Balantekin says. “We believe that some are made in supernovae explosions or neutron star mergers, and many of these objects are governed by the laws of quantum mechanics, so then you can use the stars to explore aspects of quantum mechanics.”
What is already known?
- Immediately after the Big Bang, lighter elements like hydrogen and helium were abundant. Heavier elements, up to iron (atomic number 26) continued to form through nuclear fusion in the centers of hot stars.
- Above iron, fusion is no longer energetically favorable, and nuclear synthesis occurs via neutron capture, where neutrons glom onto atomic nuclei. At high enough concentrations, neutrons can convert into protons, increasing the atomic number of the element by one.
- This conversion is dependent on neutrinos and antineutrinos. Neutron capture has been found to occur slowly (s-process, over years) and rapidly (r-process, within minutes); an intermediate timescale, or i-process has been proposed but little evidence exists to support it. Rapid or intermediate neutron capture can only take place in catastrophic events where a huge amount of energy is released, such as supernova collapse.
- “When a supernova collapse occurs, you start with a big star, which is gravitationally bound, and that binding has energy,” Balantekin says. “When it collapses, that energy has to be released, and it turns out that energy is released in neutrinos.”
- The laws of quantum mechanics state that those neutrinos can become entangled because they interact in the collapsing supernova. Entanglement is when any two or more particles interacted and then “remember” the others, no matter how far apart they might be.
A quick summary of the research
- “One question we can ask is if these neutrinos are entangled with each other or not,” Balantekin says. “This paper shows that if the neutrinos are entangled, then there is an enhanced new process of element production, the i-process.”
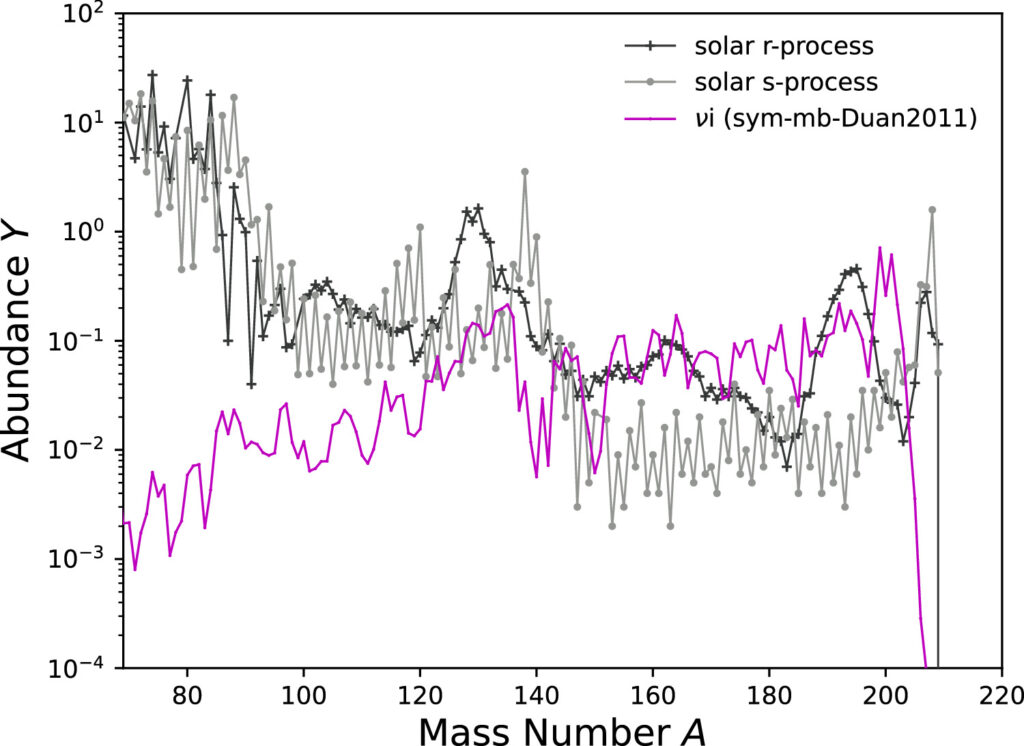
The experimental and simulated evidence
- The researchers used two known facts to set up their calculations: well-established rates of neutron capture, and catalogs of the atomic spectra of stars, which astronomers have collected over decades to identify the abundance of different elements. They also knew that a supernova collapse produces on the order of 10^58 neutrinos, a number that is far too large to use in any standard calculations.
- Instead, they made simulations of up to eight neutrinos and calculated the abundance of elements that would be created via neutron capture if the neutrinos were entangled, or were not entangled.
- “We have a system of, say, three neutrinos and three antineutrinos together in a region where there are protons and neutrons and see if that changes anything about element formation,” Balantekin says. “We calculate the abundances of elements that are produced in the star, and you see that the entangled or not entangled cases give you different abundances.”
- The simulations showed that elements with atomic number greater than 140 are likely to be enhanced by i-process neutron capture — but only if the neutrinos are entangled.
Caveats and future work
- Balantekin points out that these simulations are just “hints” based on astronomical observations. Astrophysics research requires using the cosmos as a lab, and it is difficult to conduct true experimental tests on earth.
- “There’s something called the standard model of particle physics, which determines the interaction of particles. The neutrino-neutrino interaction is one aspect of the standard model which has not been tested in the lab, it can only be tested in astrophysical extremes,” Balantekin says. “But other aspects of the standard model have been tested in the lab, so one believes that it should all work.”
- The researchers are currently using more astrophysical data of element abundance in extreme environments to see if those abundances continue to be explained by entangled neutrinos.
This research is supported in part by the National Science Foundation grants Nos. PHY-1630782 and PHY-2020275 (Network for Neutrinos, Nuclear Astrophysics and Symmetries). Balantekin is supported in part by the U.S. Department of Energy, Office of Science, Office of High Energy Physics, under Award No. DE-SC0019465 and in part by the National Science Foundation Grant PHY-2108339 at the University of Wisconsin-Madison.
The paper’s co-authors include Michael Cervia, Amol Patwardhan, Rebecca Surman, and Xilu Wang, all current or former members of N3AS.
Ellen Zweibel named Hilldale Professor
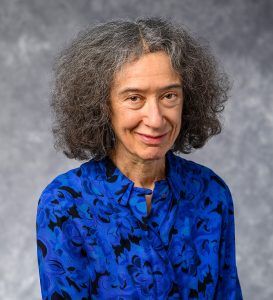
Five members of the University of Wisconsin–Madison faculty, including astronomy and physics professor Ellen Zweibel, have received Hilldale Professorships.
Hilldale Professorships are given to faculty members who excel in scholarly activity, have records of outstanding research or creative work, and show promise of continued productivity. Recipients receive a salary increase in addition to funding that may be used for research support and teaching release. Appointments are for five years with the possibility of renewal until the individual leaves the university or retires.
Zweibel’s work in theoretical astrophysicist specializes in plasma astrophysics and focuses on evolution of astrophysical magnetic fields, interstellar astrophysics, star formation, cosmic rays and stellar physics.
Ke Fang named Sloan Fellow
This story is adapted from one published by University Communications
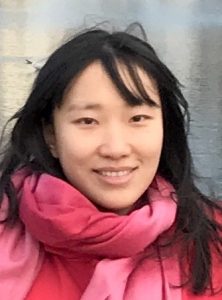
Ke Fang, assistant professor of Physics and WIPAC investigator, is among 126 scientists across the United States and Canada selected as Sloan Research Fellows.
The fellowships, awarded annually since 1955, honor exceptional scientists whose creativity, innovation and research accomplishments make them stand out as future leaders in their fields.
Using data from the Ice Cube Observatory and Fermi Large Area Telescope along with numerical simulations, Fang studies the origin of subatomic particles — like neutrinos — that reach Earth from across the universe.
“Sloan Research Fellowships are extraordinarily competitive awards involving the nominations of the most inventive and impactful early-career scientists across the U.S. and Canada,” says Adam F. Falk, president of the Alfred P. Sloan Foundation. “We look forward to seeing how fellows take leading roles shaping the research agenda within their respective fields.”
Founded in 1934, the Sloan Foundation is a not-for-profit institution dedicated to improving the welfare of all through the advancement of scientific knowledge.
Sloan Fellows are chosen in seven fields — chemistry, computer science, Earth system science, economics, mathematics, neuroscience and physics — based on nomination and consideration by fellow scientists. The 2024 cohort comes from 53 institutions and a field that included more than 1,000 nominees. Winners receive a two-year, $75,000 fellowship that can be used flexibly to advance their research.
Among current and former Sloan Fellows, 57 have won a Nobel Prize, 71 have been awarded the National Medal of Science, 17 have won the Fields Medal in mathematics and 23 have won the John Bates Clark Medal in economics.
Xiangyao Yu, assistant professor of computer sciences at UW–Madison, was also named a Sloan Fellow.