Elements are the building blocks of every chemical in the universe, but how and where the different elements formed is not entirely understood. A new paper in The Astrophysical Journal by University of Wisconsin–Madison physics professor Baha Balantekin and colleagues with the Network for Neutrinos, Nuclear Astrophysics, and Symmetries (N3AS) Physics Frontier Center, shows how entangled neutrinos could be required for the formation of elements above approximately atomic number 140 via neutron capture in an intermediate-rate process, or i-process.
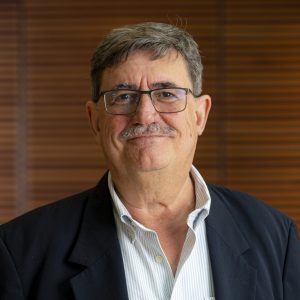
Why it’s important
“Where the chemical elements are made is not clear, and we do not know all the possible ways they can be made,” Balantekin says. “We believe that some are made in supernovae explosions or neutron star mergers, and many of these objects are governed by the laws of quantum mechanics, so then you can use the stars to explore aspects of quantum mechanics.”
What is already known?
- Immediately after the Big Bang, lighter elements like hydrogen and helium were abundant. Heavier elements, up to iron (atomic number 26) continued to form through nuclear fusion in the centers of hot stars.
- Above iron, fusion is no longer energetically favorable, and nuclear synthesis occurs via neutron capture, where neutrons glom onto atomic nuclei. At high enough concentrations, neutrons can convert into protons, increasing the atomic number of the element by one.
- This conversion is dependent on neutrinos and antineutrinos. Neutron capture has been found to occur slowly (s-process, over years) and rapidly (r-process, within minutes); an intermediate timescale, or i-process has been proposed but little evidence exists to support it. Rapid or intermediate neutron capture can only take place in catastrophic events where a huge amount of energy is released, such as supernova collapse.
- “When a supernova collapse occurs, you start with a big star, which is gravitationally bound, and that binding has energy,” Balantekin says. “When it collapses, that energy has to be released, and it turns out that energy is released in neutrinos.”
- The laws of quantum mechanics state that those neutrinos can become entangled because they interact in the collapsing supernova. Entanglement is when any two or more particles interacted and then “remember” the others, no matter how far apart they might be.
A quick summary of the research
- “One question we can ask is if these neutrinos are entangled with each other or not,” Balantekin says. “This paper shows that if the neutrinos are entangled, then there is an enhanced new process of element production, the i-process.”
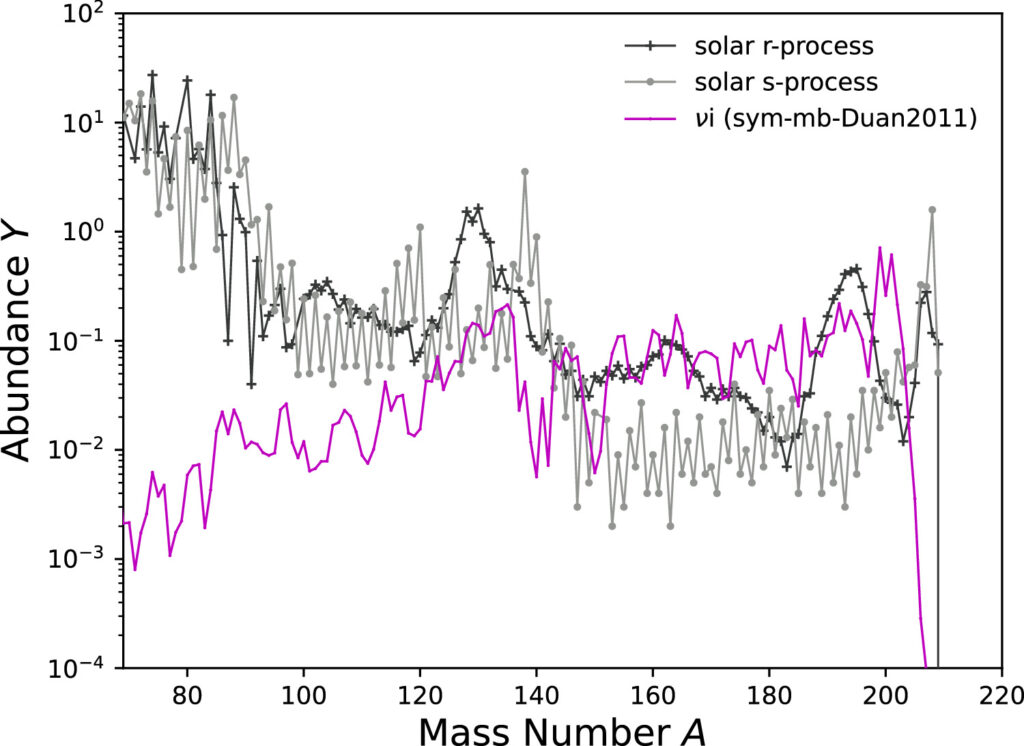
The experimental and simulated evidence
- The researchers used two known facts to set up their calculations: well-established rates of neutron capture, and catalogs of the atomic spectra of stars, which astronomers have collected over decades to identify the abundance of different elements. They also knew that a supernova collapse produces on the order of 10^58 neutrinos, a number that is far too large to use in any standard calculations.
- Instead, they made simulations of up to eight neutrinos and calculated the abundance of elements that would be created via neutron capture if the neutrinos were entangled, or were not entangled.
- “We have a system of, say, three neutrinos and three antineutrinos together in a region where there are protons and neutrons and see if that changes anything about element formation,” Balantekin says. “We calculate the abundances of elements that are produced in the star, and you see that the entangled or not entangled cases give you different abundances.”
- The simulations showed that elements with atomic number greater than 140 are likely to be enhanced by i-process neutron capture — but only if the neutrinos are entangled.
Caveats and future work
- Balantekin points out that these simulations are just “hints” based on astronomical observations. Astrophysics research requires using the cosmos as a lab, and it is difficult to conduct true experimental tests on earth.
- “There’s something called the standard model of particle physics, which determines the interaction of particles. The neutrino-neutrino interaction is one aspect of the standard model which has not been tested in the lab, it can only be tested in astrophysical extremes,” Balantekin says. “But other aspects of the standard model have been tested in the lab, so one believes that it should all work.”
- The researchers are currently using more astrophysical data of element abundance in extreme environments to see if those abundances continue to be explained by entangled neutrinos.
This research is supported in part by the National Science Foundation grants Nos. PHY-1630782 and PHY-2020275 (Network for Neutrinos, Nuclear Astrophysics and Symmetries). Balantekin is supported in part by the U.S. Department of Energy, Office of Science, Office of High Energy Physics, under Award No. DE-SC0019465 and in part by the National Science Foundation Grant PHY-2108339 at the University of Wisconsin-Madison.
The paper’s co-authors include Michael Cervia, Amol Patwardhan, Rebecca Surman, and Xilu Wang, all current or former members of N3AS.