Research
Qolab, the first UW–Madison-incubated quantum startup, joins the Chicago Quantum Exchange
U.S. Cyber Command visit highlights UW–Madison’s leadership in cyber research and education
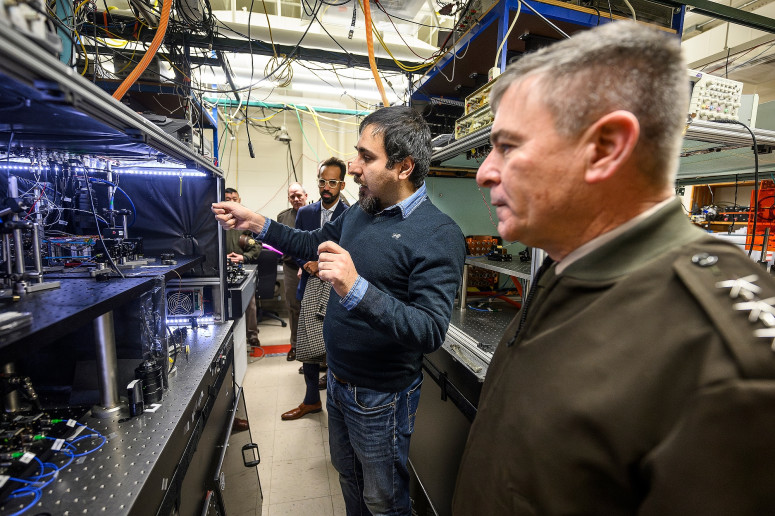
UW–Madison plays a leading role as a research and education partner for national cybersecurity. It reinforced this commitment recently by welcoming to campus a delegation from the United States Cyber Command (USCYBERCOM), which is responsible for the Department of Defense’s cyberspace capabilities.
Read the full article at: https://news.wisc.edu/u-s-cyber-command-visit-highlights-uw-madisons-leadership-in-cyber-research-and-education/HAWC detection of an ultra-high-energy gamma-ray bubble around a microquasar
This story is adapted from the HAWC Collaboration press release. Microquasars—compact regions surrounding a black hole with a mass several times that of its companion star—have long been recognized as powerful particle accelerators within our galaxy. The enormous jets spewing out of microquasars are thought to play an important role in the production of galactic cosmic rays, although [...]
Read the full article at: https://wipac.wisc.edu/hawc-detection-of-an-ultra-high-energy-gamma-ray-bubble-around-a-microquasar/First plasma marks major milestone in UW–Madison fusion energy research
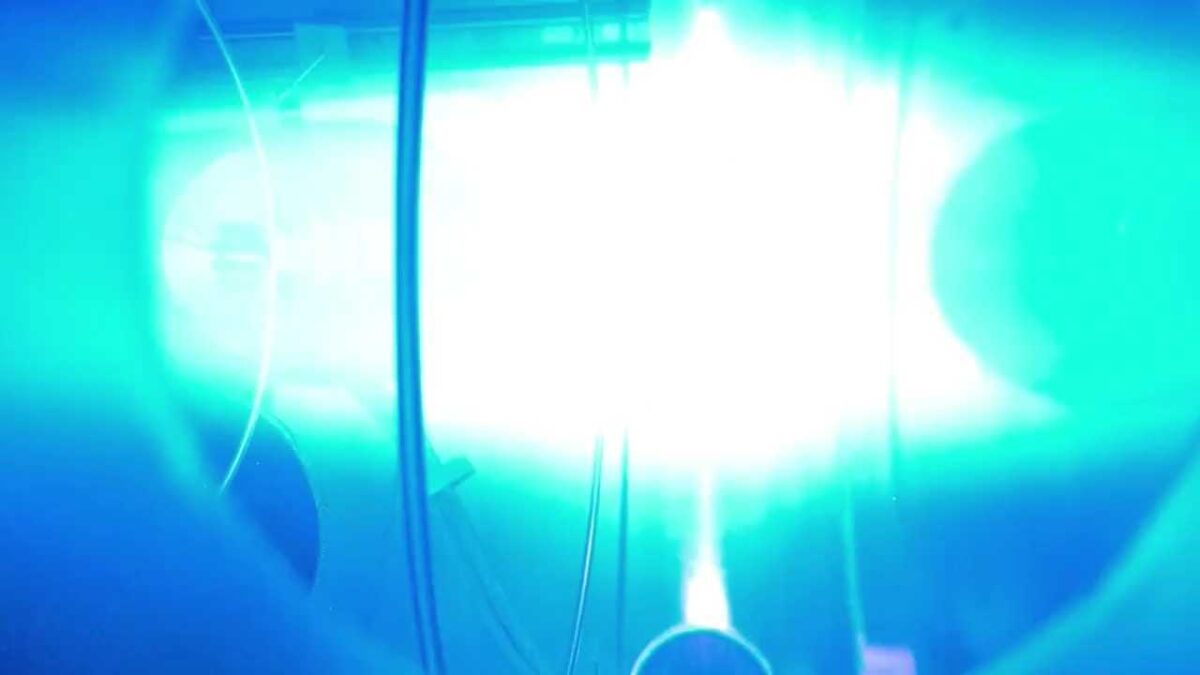
A fusion device at the University of Wisconsin–Madison generated plasma for the first time Monday, opening a door to making the highly anticipated, carbon-free energy source a reality.
Over the past four years, a team of UW–Madison physicists and engineers has been constructing and testing the fusion energy device, known as WHAM (Wisconsin HTS Axisymmetric Mirror) in UW’s Physical Sciences Lab in Stoughton. It transitioned to operations mode this week, marking a major milestone for the yearslong research project that’s received support from the U.S. Department of Energy.
“The outlook for decarbonizing our energy sector is just much higher with fusion than anything else,” says Cary Forest, a UW–Madison physics professor who has helped lead the development of WHAM. “First plasma is a crucial first step for us in that direction.”
WHAM started in 2020 as a partnership between UW–Madison, MIT and the company Commonwealth Fusion Systems. Now, WHAM will operate as a public-private partnership between UW–Madison and spinoff company Realta Fusion Inc., positioning it as major force for fusion research advances at the university.
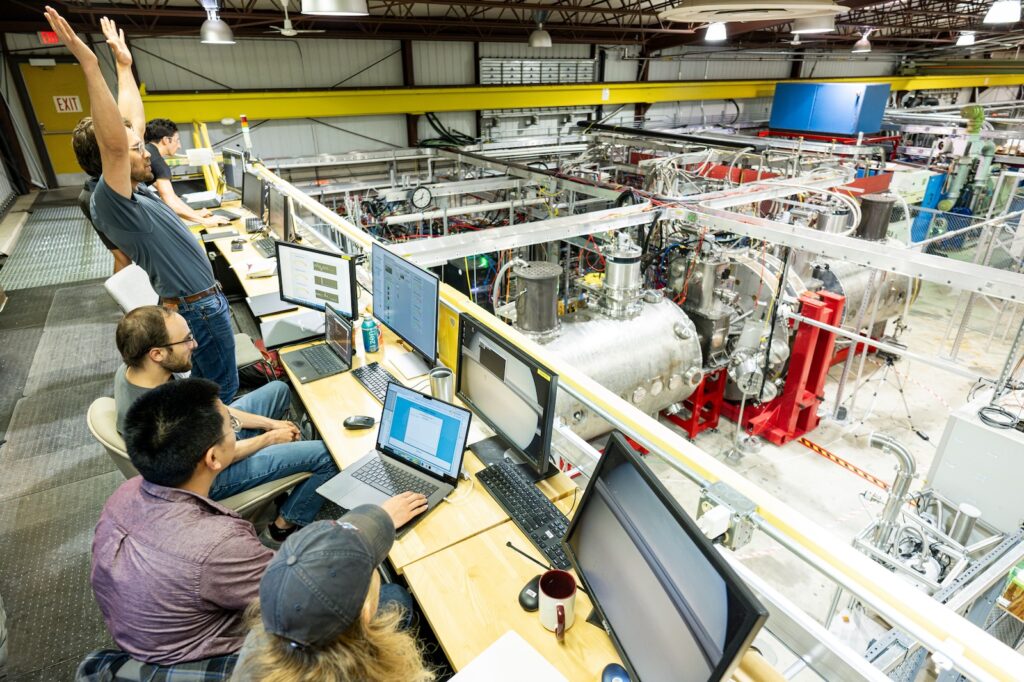
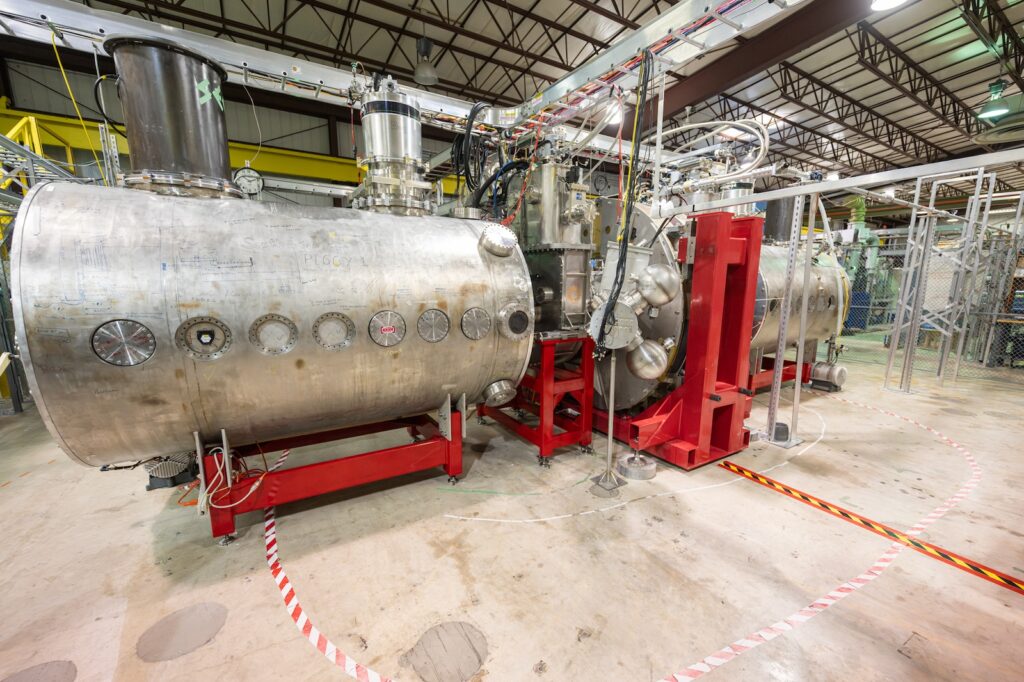
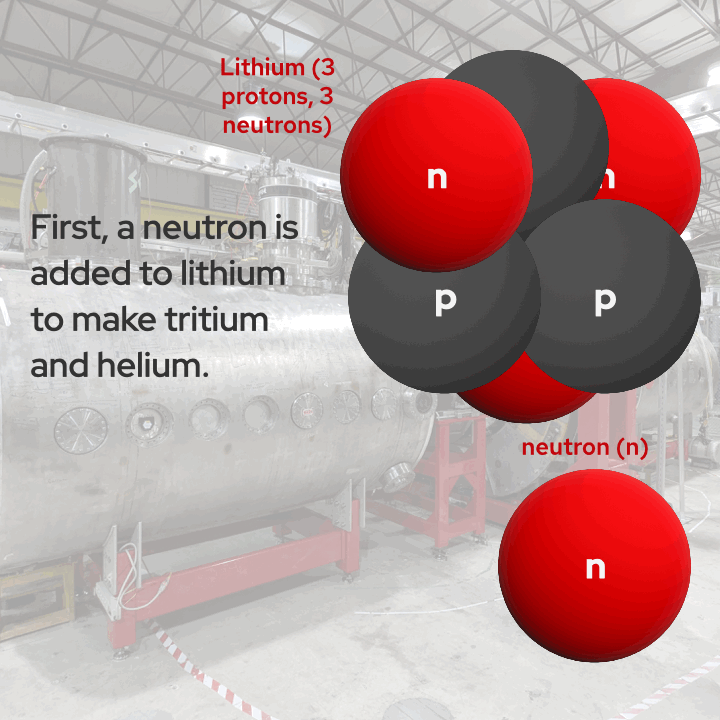
The largest magnetic fields in galaxy clusters have been revealed for the first time
By Alex Lazarian, Yue Hu, and Ka Wai Ho
Galaxy clusters, immense assemblies of galaxies, gas, and elusive dark matter, form the cornerstone of our Universe’s grandest structure — the cosmic web. These clusters are not just gravitational anchors, but dynamic realms profoundly influenced by magnetism. The magnetic fields within these clusters are pivotal, shaping the evolution of these cosmic giants. They orchestrate the flow of matter and energy, directing accretion and thermal flows, and are vital in accelerating and confining high-energy charged particles/cosmic rays.
However, mapping the magnetic fields on the scale of galaxy clusters posed a formidable challenge. The vast distances and complex interactions with magnetized and turbulent plasmas diminish the polarization signal, a traditionally used informant of magnetic fields. Here, the groundbreaking technique — synchrotron intensity gradients (SIG) — developed by a team of UW–Madison astronomers and physicists led by astronomy professor Alexandre Lazarian, marks a turning point. They shifted the focus from polarization to the spatial variations in synchrotron intensity. This innovative approach peels back layers of cosmic mystery, offering a new way to observe and comprehend the all-important magnetic tapestry on scale of millions of light years.
A landmark study published in Nature Communications has employed the SIG technique to unveil the enigmatic magnetic fields within five colossal galaxy clusters, including the monumental El Gordo cluster, observed with the Very Large Array (VLA) and MeerKAT telescope. This colossal cluster, formed 6.5 billion years ago, represents a significant portion of cosmic history, dating back to nearly half the current age of the universe. The findings in El Gordo, characterized by the largest magnetic fields observed, provide crucial insights into the structure and evolution of galaxy clusters.
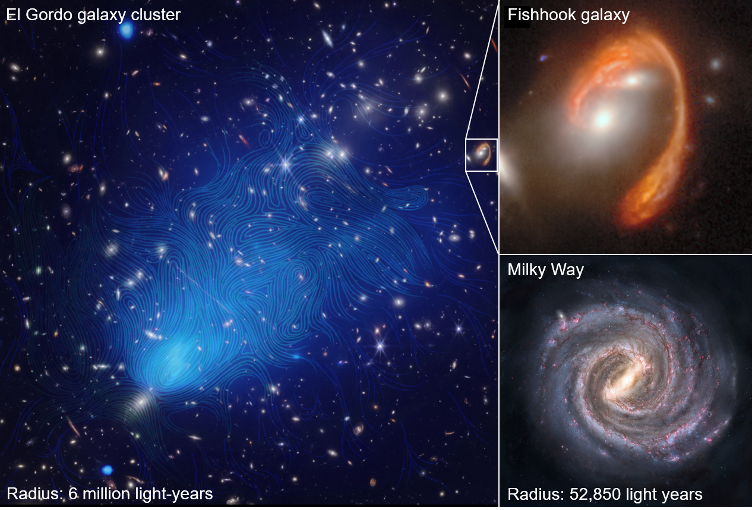
The research is a fruitful collaboration between the UW–Madison team and their Italian colleagues, including Gianfranco Brunetti, Annalisa Bonafede, and Chiara Stuardi from the Instituto do Radioastronomia (Bologna, Italy) and the University of Bologna. Brunetti, a renowned expert in the high-energy physics of galaxy clusters, is enthusiastic about the potential that the SIG technique holds for exploring magnetic field structures on even larger scales, such as the Megahalos recently discovered by him and his colleagues.
Echoing this excitement is the study’s lead researcher, physics graduate student Yue Hu.
“This research marks a significant milestone in astrophysics,” Hu says. “Utilizing the SIG method, we’ve observed and begun to comprehend the nature of magnetic fields in galaxy clusters for the first time. This breakthrough heralds new possibilities in our quest to unravel the mysteries of the universe.”
This study lays the groundwork for future explorations. With the SIG method’s proven effectiveness, scientists are optimistic about its application to even larger cosmic structures that have been detected recently with the Square Kilometre Array (SKA), promising deeper insights into the mysteries of the Universe magnetism and its effects on the evolution of the Universe Large Scale Structure.
Earth-sized planet discovered in ‘our solar backyard’
A team of astronomers have discovered a planet closer and younger than any other Earth-sized world yet identified. It’s a remarkably hot world whose proximity to our own planet and to a star like our sun mark it as a unique opportunity to study how planets evolve.
The new planet was described in a new study published this week by The Astronomical Journal. Melinda Soares-Furtado, a NASA Hubble Fellow at the University of Wisconsin–Madison who will begin work as an astronomy and physics professor at the university in the fall, and recent UW–Madison graduate Benjamin Capistrant, now a graduate student at the University of Florida, co-led the study with co-authors from around the world.
“It’s a useful planet because it may be like an early Earth,” says Soares-Furtado.
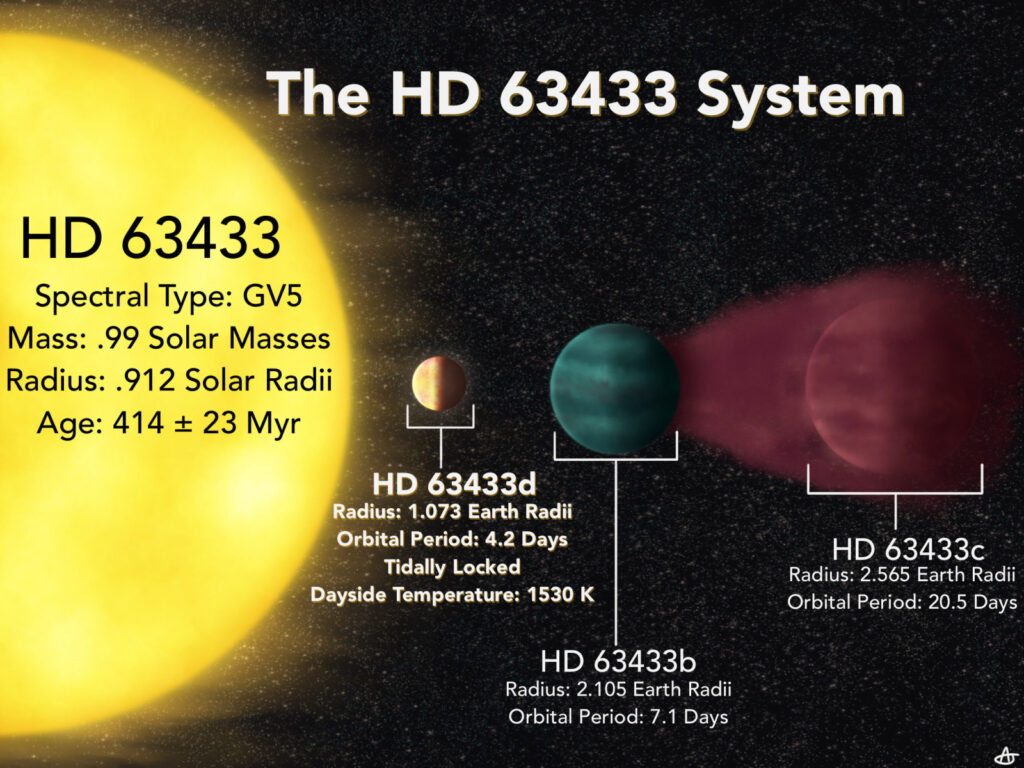
A new spin on an old superconductor means that it can be an ideal spintronic material, too
Back in the 1980s, researchers discovered that a bismuthate oxide material was a rare type of superconductor that could operate at higher temperatures. Now, a team of engineers and physicists at the University of Wisconsin-Madison has found the material, “Ba(Pb,Bi)O3,” is unique in another way: It exhibits extremely high spin orbit torque, a property useful in the emerging field of spintronics.
The combination makes this and similar materials potentially important in developing the next generation of fast, efficient memory and computing devices.
The finding was an encouraging surprise to Chang Beom-Eom, a professor of materials science and engineering, and Mark Rzchowski, a professor of physics, both at UW-Madison. “We’re looking to expand the range of materials that can be used in spintronic applications,” says Rzchowski. “We had known from previous work these oxides have a lot of interesting properties, and so were investigating the spintronic characteristics. We weren’t anticipating such a large effect. The origins of this are not theoretically understood, but we can speculate about some interesting physical mechanisms.”
The paper was published Dec. 5, 2023, in the journal Nature Electronics.
In conventional electronics, positive and negative electric charges are used to flip millions or billions of tiny transistors on semiconductor chips or in memory devices. But in spintronics, magnetic fields, and interactions with other electrons, manipulate a fundamental property of electrons called the spin state, which records information. This is much faster, more energy-efficient and more powerful than current semiconductors and will advance the development of quantum computing and low-power devices.
Featured image caption: Chang Beom-Eom, a professor of materials science and engineering, and Mark Rzchowski, a professor of physics, in the lab. Photo: Joel Hallberg.
IceCube shows Milky Way galaxy is a neutrino desert
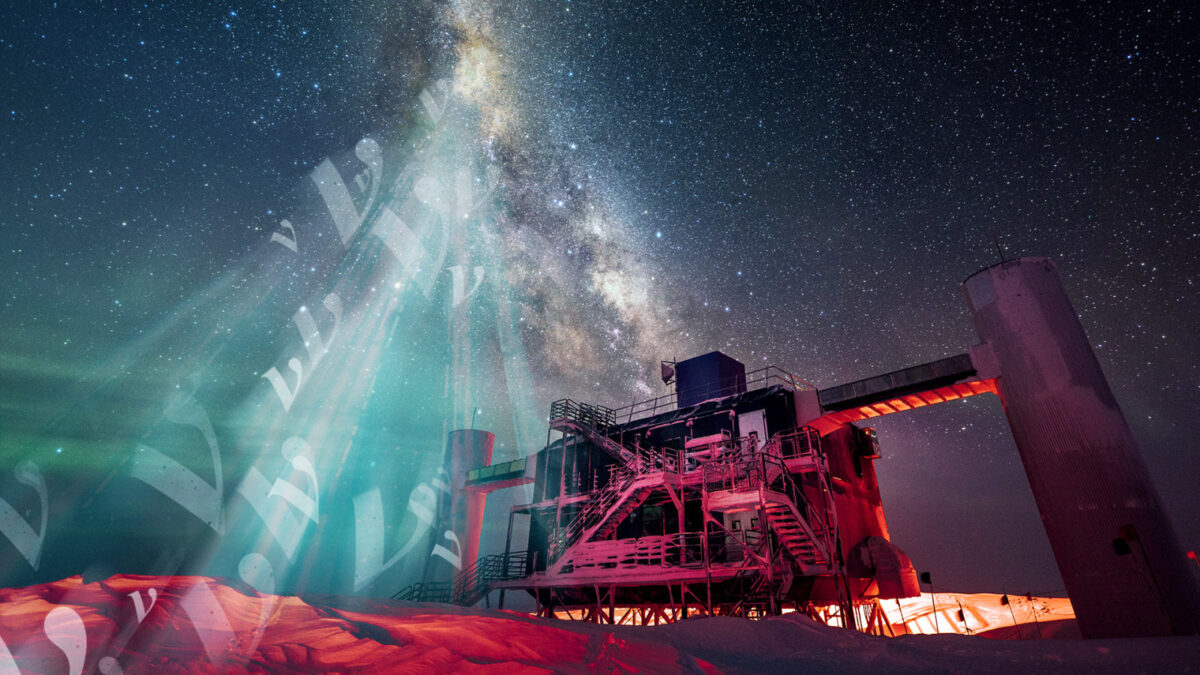
The Milky Way galaxy is an awe-inspiring feature of the night sky, dominating all wavelengths of light and viewable with the naked eye as a hazy band of stars stretching from horizon to horizon. Now,
In a June 30 article in the journal Science, the IceCube Collaboration — an international group of more than 350 scientists — presents this new evidence of high-energy neutrino emission from the Milky Way. The findings indicate that the Milky Way produces far fewer neutrinos than the average distant galaxies.
“What’s intriguing is that, unlike the case for light of any wavelength, in neutrinos, the universe outshines the nearby sources in our own galaxy,” says Francis Halzen, a professor of physics at the University of Wisconsin–Madison and principal investigator at IceCube.
The IceCube search focused on the southern sky, where the bulk of neutrino emission from the galactic plane is expected near the center of the galaxy. However, until now, a background of neutrinos and other particles produced by cosmic-ray interactions with the Earth’s atmosphere made it difficult to parse out neutrinos originating from galactic sources — a significant challenge compounded by relatively sparse neutrino production in general.
Two students receive Sophomore Research Fellowships
Two physics majors have received a UW–Madison Sophomore Research Fellowship as a fellow or honorable mention. The students are:
- Erica Magee, Mathematics, Physics; working with Martin Zanni (Chemistry)
- Elias Mettner, Physics; working with Abdollah Mohammadi (Physics)
The fellowships include a stipend to each student and to their faculty advisers. Fellowships are funded by grants from the Brittingham Wisconsin Trust and the Kemper K. Knapp Bequest, with additional support from UW-System, the Chancellor’s Office and the Provost’s Office.