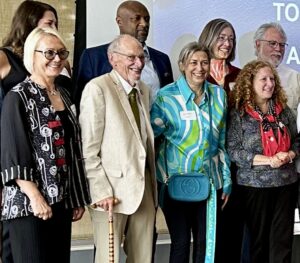
Back row from left: Lauren Bishop (professor of Social Work, Romnes winner), Charles Lee Isbell, Jr. (UW Provost), Susan Harness (Maria Stuchly Professor of Electrical Engineering), Russ Shafer-Landau (Elliott Sober Professor of Philosophy).
Physics professor Pupa Gilbert is one of eight UW–Madison faculty awarded WARF Named Professorships, which come with $100,000 and honor faculty who have made major contributions to the advancement of knowledge, primarily through their research endeavors, but also as a result of their teaching and service activities. Award recipients choose the names associated with their professorships.
Gilbert, the John D. Wiley professor of physics, joined the Department of Physics in 1999 as a full professor, following staff scientist positions in the Italian National Research Council (CNR) in Rome and the Swiss Institute of Technology in Lausanne (EPFL). Her research focuses on biomineralization, that is, on understanding the nanoscale formation mechanisms of natural biominerals, especially coral skeletons, but on a side she also studies sea urchin spines, mollusk shell nacre, tooth enamel, and inner ear cochleaer bone. She also strives to understand the evolution of biomineralization, and how biominerals interact with the environment in the past, present, and future. She is actively working to save coral reefs from climate change.
Her role in the profession has included being research director for the NSF-funded Synchrotron Radiation Center at UW–Madison, chair of the Division of Biological Physics (DBIO) of the American Physical Society, a Radcliffe Fellow at Harvard University, and establishing “the Cnidarians,” a group of tens of diverse undergraduate students at UW–Madison who produce great discoveries by doing massively parallel data processing. She teaches Physics in the Arts, a popular course for non-science majors on light, color, and sound. She was knighted by Italian President Carlo A. Ciampi in 2001.
“Prof. Pupa Gilbert is a luminary in the field of biomineralization, developing a fundamental understanding of these crucial processes that are shared by many diverse organisms,” says Kevin Black, chair of the UW–Madison department of physics. “Prof. Gilbert is a knight of the Italian Republic, and stalwart advocate for involving undergraduates, in particular those from underrepresented groups in her research. This award recognizes the many contributions to the academy in her long and productive interdisciplinary research program and is well deserved.”
For her named professorship, Gilbert chose John D. Wiley, the former UW–Madison Chancellor who earned his MS and PhD from this department. He was a member of the technical staff at Bell Laboratories, then a visiting scientist at Max Planck Institute for Semiconductor Physics. He joined the faculty at UW–Madison as professor of electrical and computer engineering in 1975, where he did groundbreaking research on semiconductors and metals, and earned major patents at WARF. Later, he became Chair of the Materials Science Program, Dean of the Graduate School, Provost, and then Chancellor (2001-2008), all at UW–Madison.
Wiley also started campus-wide initiatives such as cluster hiring and originated many campus building projects. He was the first Director of the Wisconsin Institute of Discovery, a position he held from 2008 until he retired in 2011.
Gilbert chose Wiley, whom she calls “a brilliant physicist,” for her named professorship for all the reasons listed above. She also credits him with bringing her to UW–Madison.
“When there was a cluster hire in biophotonics back in 1999, John Wiley wanted me to join the university and he had them include the word spectromicroscopy — the synchrotron method that I developed — into the search to encourage me to apply,” Gilbert says. “I really didn’t want to apply, I was on my way to CalTech, but of course I couldn’t say no to John.”
In total, thirty-two University of Wisconsin–Madison faculty were awarded fellowships from the Office of the Vice Chancellor for Research for 2024-25, including eight WARF named professorships, 13 Romnes faculty fellowships, and 11 Kellett mid-career awards. The awardees span the four research divisions on campus: arts and humanities, physical sciences, social sciences and biological sciences.
“These awards recognize excellence in faculty research, academics, and outreach at various stages of their scholarly careers and provide an opportunity for continued development of their research programs,” says Cynthia Czajkowski, interim vice chancellor for research. “I look forward to seeing the results of their imaginative use of these funds.”
The awards are possible due to the research efforts of UW–Madison faculty and staff. Technology that arises from these efforts is licensed by the Wisconsin Alumni Research Foundation and the income from successful licenses is returned to the OVCR, where it’s used to fund research activities and awards throughout the divisions on campus.